Archaean supercontinents

(image at left – current approximate distribution of most Archean crustal rocks (the projection greatly exaggerates the area of polar landmasses – compare with image of location of cratons and platforms)
The Archean mantle was hotter than the Earth's current interior, resulting in heavy mantle convection and crustal turbulence. Archean Earth was very active tectonically, with higher rates of volcanic activity and plate boundary movement than is occurring today. Active tectonics during the Archean created numerous, mobile protocontinental landmasses that floated on the turbulent mantle. Toward the end of the Archean, the proto-continents began to coalesce, and by about 3.3 Ga the more tectonically stable supercontinent Vaalbara is hypothesized to have formed by accretion of smaller landmasses.
The oldest Archaean rocks so far discovered on Earth are:
1) The Isua Supracrustal rocks of West Greenland (3.7 to 3.8 Ga)
2) Northern Michigan (3.5-3.7 Ga)
3) Swaziland (3.4-3.5 Ga)
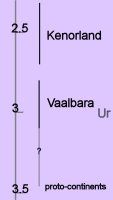
70% of modern continental shields date from 3.0 to 2.5 billion years ago, which was the period of maximum continent formation. Most of the earliest rocks have been greatly altered through regional metamorphic processes, but later rocks (3.2-2.5 Ga) are mostly pillow-basalts that formed beneath the vast oceans. Archean sedimentary rocks are mostly coarse and poorly sorted sandstones and conglomerates.
The oldest fossilized signs of life date from the Archean – Cyanobacteria that created 3.45 Ga old stromatolite fossils found in the Apex Chert at Marble Bar, Western Australia. Microfossils from Swaziland (South Africa) have a similar age.[image]
Vaalbara is Earth's theorized first supercontinent, which, according to radiometric data, existed by 3.3 billion years ago (3.3 Ga) and possibly even as far back as 3.6 Ga. Evidence includes geochronological and palaeomagnetic studies between the two Archaean cratons (protocontinents) called the Kaapvaal craton (the Kaapvaal province of South Africa) and the Pilbara craton (the Pilbara province of Western Australia).
Further evidence is the structural sequence similarities of the greenstone belts and gneiss belts of the Kaapvaal and Pilbara cratons. These same Archaean greenstone belts are now spread out across the margins of the Superior craton of Canada and across the cratons of the former Gondwana and Laurasia continents. The subsequent drift paths of the Kaapvaal and Pilbara cratons after 2.8 Ga gives further evidence that they were once connected.
It is not certain when Vaalbara began to break up, but geochronological and palaeomagnetic evidence show that the two cratons had a rotational 30 degree latitudinal separation at 2.78 to 2.77 Ga, implying that they were no longer contiguous after ~2.8 Ga.
Ur was the first known continent, which probably formed 3 billion years ago in the early Archean Eon. Ur joined with the continents Nena and Atlantica about one billion years ago to form the supercontinent Rodinia. Ur survived for a long time, until it was torn apart when the supercontinent Pangaea broke apart about 208 million years ago into Laurasia and Gondwanaland. Ur now forms parts of Africa, Australia, India, and Madagascar. In the early period of its existence, it was probably the only continent on Earth, and is so considered by some to be a supercontinent, even though it was probably smaller than Australia is now.
By about 2.7 Ga, Neoarchean sanukitoid cratons plus new continental crust accreted to form another of Earth's earliest supercontinents, Kenorland, which comprised the Laurentia, Baltica, Australia, and Kalahari cratons. The Baltic/Fennoscandian Shield formed the core of Kenorland, with origins that trace back more than 3.1 Ga. The Yilgarn Craton (present-day Western Australia) contains 4.4 Ga detrital zircon elements.
Protracted tectonic magna plume rifting occurred between 2.48 to 2.10 Ga, contributing to the break-up of Kenorland during the Late Neoarchaean and early Paleoproterozoic Era (2.48 to 2.10 Ga) during the Siderian and Rhyacian periods. The rifting is manifested by mafic dykes and sedimentary rift-basins and rift-margins on many continents. On early Earth, this type of bimodal deep mantle plume rifting was common in Archaean and Neoarchaean crust and continent formation.
The geological time period remains conjectural at which Earth made the transition from deep-mantle-plume mechanism of continent formation to the current two-layer core-mantle plate tectonics convection mechanism that forms continents by subduction, convergence and accretion. However, with the findings of the earlier continent Ur and the ca. 3.1 Ga supercontinent Vaalbara, this transition period may have occurred much than the time of Kenorland.
Paleomagnetic studies indicate that Kenorland lay in low latitudes until the rifting breakup commenced around 2.48 Ga. Paleomagnetic evidence further indicates that at 2.45 Ga the Baltic/Fennoscandian Shield, astride the equator, was attached to Laurentia (the Canadian Shield), forming a unity with both the Kola and Karelia cratons, which began to drift apart ~2.45 Ga. By 2.4 Ga, the Kola craton was located at ~15 degrees latitude and the Karelia craton had drifted to ~30 degrees latitude. Paleomagnetic evidence indicates that at 2.45 Ga the Yilgarn craton (now the bulk of Western Australia) was not connected to Fennoscandia-Laurentia and was located at ~70 degrees latitude. This suggests that Kenorland was no longer a supercontinent by 2.45 Ga. By 2.4 Ga, an ocean separated the formerly joined Kola and Karelia cratons.
Based on the rift margin spatial arrangements of Laurentia, it is hypothesized that the Slave and Superior cratons were not part of the rifting supercontinent Kenorland, but may instead have been two different Neoarchaean landmasses (supercratons) located at opposite poles of a very large Kenorland. This speculation is based on the mechanics of how drifting assemblies of various constituent pieces should flow reasonably together toward the amalgamation of the new subsequent continent. The Slave and Superior cratons now constitute the NW and SE portions of the Canadian Shield, respectively.
The breakup of Kenorland was contemporary with the 60 million year long Huronian glaciation. The banded iron formations (BIF) show their greatest extent at this period, indicating massive increase in oxygen build-up (estimated 0.1% to 1% of atmosphere). Rising oxygen levels caused virtual disappearance of the greenhouse gas methane, which was oxidized into carbon dioxide and water. The simultaneous breakup of Kenorland increased continental rainfall, increasing erosion and further reducing the other greenhouse gas carbon dioxide. This reduction in greenhouse gases coupled with solar output still less than 85% its current levels precipitated a runaway Snowball Earth scenario, when average temperatures planet-wide plummeted to below freezing. Despite the anoxia indicated by the BIF, photosynthesis continued, stabilizing climates at new levels during the second part of the Proterozoic Era.
◙ subduction zone magmas ◙
More: GeoWhen Database : Tectospheric keels : 2004 time scale endorsed by the International Commission on Stratigraphy
Labels: Archean Eon, banded iron formation, glaciation, gneiss belt, Gondwana, Kaapvaal, Kenorland, Laurasia, paleomagnetic, Pangea, Pilbara, radiometric, Rodinia, Snowball Earth, Ur, Vaalbara supercontinent

