A
geologic province is an extensive region with distinctive geologic attributes that differentiate it from surrounding areas. A province may include a single dominant structural element (basin, fold belt) or a number of contiguous related elements. Adjoining provinces may be similar in structure but be considered separate due to differing histories.
Above, left: Map of world geologic provinces
Oceanic crust █ 0-20 Ma
█ 20-65 Ma
█ >65 Ma
Geologic province █ Shield █ Platform █ Orogen █ Basin █ Large igneous province █ Extended
crust :
Source :
hi-res image :
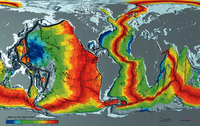
The
continental crust is composed of lower density, more ancient
felsic rocks of different composition than the
mafic rocks of the
oceanic crust, the oldest of which are about 180 million years old. Oceanic rocks arise in the oceanic ridge system (left - click to enlarge,
hi-res).
∎ A
shield is defined as an extensive area of very ancient rocks that have been
exposed and levelled by erosion (peneplanation). The exposed
Precambrian rocks of a shield are crystalline igneous and high-grade metamorphic rocks within tectonically stable areas lacking active orogenic belts.
On a shield the (usually) Precambrian basement rocks of the craton crop out extensively at the surface. A
craton is an extensive portion of a continental plate that has remained relatively
undisturbed since the Precambrian era, and includes
both shield and platform layers.
∎ A
platform is that portion of a continent that is
covered by horizontal or gently tilted rock and is underlain by very ancient rocks of the crystalline basement. The ancient bedrock was consolidated during deformations that preceded deposition of the sedimentary rocks of the overlying platform layer.
∎ An
orogenic belt is that part of the continent where
deformation of the Earth’s
crust created a
mountain range, which may since have eroded down to the roots of the original mountains.
Because
shield areas have been little affected by any
tectonic events since the
Precambrian, they are relatively
flat regions in which mountain building, faulting, and other tectonic processes are greatly diminished compared to the activity occuring at shield
margins and at the boundaries between tectonic plates. Because of their stability, shields have been flattened by erosion; however, shields commonly have a very gently convex surface and are surrounded by sediment covered platforms. Together, the
shield,
platform and
basement comprise the
craton.
Shields are very
complex, comprising vast areas of
granitic or
granodioritic gneisses, usually of
tonalitic composition. They contain belts of
sedimentary rocks, often surrounded by low-grade
volcano-
sedimentary greenstone belt sequences containing metamorphosed
greenschist,
amphibolite, and
granulite facies. The marginal areas of stable shields often display complex
orogenic sequences occuring during the past few hundred million years. Shield margins generally exhibit tectonic or plate-like dynamic mechanisms.
Shields form the nucleus of most continents and typically are bordered by
orogenic belts of folded Cambrian rocks. Continents have expanded through
accretion of younger rocks that have undergone deformations through successions of mountain building episodes. Thus, belts of folded rocks were welded onto the borders of the preexisting shields, increasing the area of proto-continents. The margins of shield are subjected to geotectonic forces that remodel the
cratons that they partially comprise.
∎ A
structural basin is a large-scale formation of
rock strata deformed by
tectonic warping of previously flat lying strata. Structural basins have affinities with geological depressions, but are distinct from sedimentary basins in which time-dependant aggregation of rocks has in-filled a depression or accumulated within an area. Downwarped strata form
synclines, which are concave geological
folds, with layers that dip downward toward the center of the structure.
Basins can develop through greater erosion of the fractured, upwarped rocks at the center of an
anticline, such that strata that originally lay at the top of the dome are eroded most. Such structural basins are the inverse of
domes, which are symmetrically-dipping
anticlines. Because a structural basin's strata dip toward the center, the exposed strata in a basin have the youngest rocks in the center.
Oceanic basins commence at continental shorelines, but each continent extends under the adjacent ocean. The
continental shelf (or
continental platform) comprises the extension of a continent under an ocean. A continental shelf is characterized by a very gentle slope and generally reaches depths of less than 200 metres, at which point there is a steep slope down to the
ocean floor.
Shields:African, or Ethiopian Shield
Amazonian Shield of central South America
The Angaran Shield of West Siberia
Arabian-Nubian Shield
(Western) Australian Shield
Baltic Shield of Scandinavia and Eastern Europe
Canadian Shield AKA
Laurentian ShieldThe China-Korean Shield containing the North China Craton
The East Antarctic Shield containing the East Antarctic craton
Guiana Shield
Indian Shield
Labels: amphibolite, basin, continental crust, craton, geologic province, granulite facies, greenschist, large igneous province, Oceanic crust, orogen, Platform, Precambrian rocks, Shield
|
